Meiosis
Cells containing two sets of chromosomes, like those created during mitosis, are called diploid. However, during the fertilization required for sexual reproduction, two reproductive cells from two individual organisms are joined. If those cells (a sperm cell and an egg cell, also called gametes) each contained two sets of chromosomes, the resulting cell would have four sets. In order to prevent this doubling of chromosomes, the diploid cell must somehow reduce its number of chromosome sets before fertilization can occur again. This is accomplished via the process of meiosis.
Diploid cells that contain two copies of each chromosome are known as homologous chromosomes: matched pairs containing the same genes in identical locations along their length. As you have learned, mitosis is the part of the cell cycle that results in identical daughter cells that are also genetically identical to the original parent cells. This means that homologous chromosomes are duplicated, so that each new cell receives a complete, identical set.
Haploid cells, containing a single copy of each homologous chromosome, are found only within structures that give rise to gametes. The nuclear division that forms haploid cells is called meiosis, and employs many of the same mechanisms as mitosis. However, the starting nucleus is always diploid and the nuclei that result at the end of a meiotic cell division are haploid. To achieve this reduction in chromosome number, meiosis consists of one round of chromosome duplication and two rounds of nuclear division.
Because the events that occur during each of the division stages are analogous to the events of mitosis, the same stage names are assigned. However, because there are two rounds of division, the major process and the stages are designated with a “I” or a “II.” Thus, meiosis I is the first round of meiotic division and consists of prophase I, and so on. Meiosis II, in which the second round of meiotic division takes place, includes prophase II, and so on.
Meiosis is preceded by an interphase consisting of the G1, S, and G2 phases, which are nearly identical to the phases preceding mitosis. During DNA duplication in the S phase, each chromosome is replicated to produce two identical copies, called sister chromatids, that are held together at a structure called a centromere until anaphase II.
Meiosis I
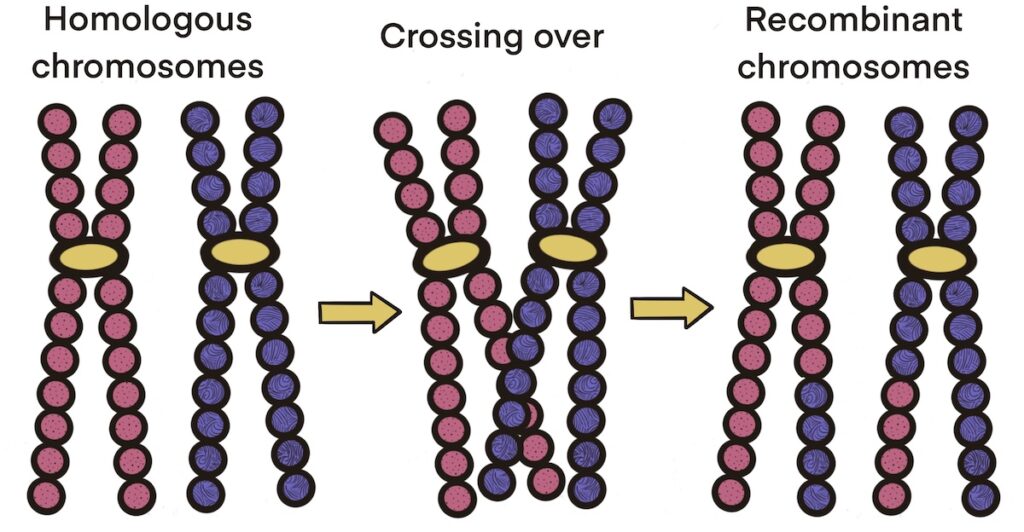
Early in Prophase I, as the nuclear envelope begins to break down, homologous chromosomes pair up with one another so genes are aligned precisely with each other. As in mitosis, spindle fibers from centrosomes will attach to the centromeres, but in this case they attach to a set of homologous chromosomes. While the chromosomes are lined up in this way, a process called crossing over can take place, in which chromosomal segments from maternal DNA can be exchanged with paternal DNA. The crossover events are the first source of genetic variation in the nuclei produced by meiosis. When sister chromatids are separated, each will carry some DNA from one parent of the individual and some DNA from the other parent. Each recombinant chromatid has a combination of maternal and paternal genes that did not exist before the crossover.
During Metaphase I, homologous chromosomes are arranged randomly along the equator of the cell. This is important in determining the genes that will be passed along to the final daughter cell, as each will only receive one of the two homologous chromosomes. This randomness is the physical basis for the creation of the second form of genetic variation in offspring. Because there is an equal chance that a spindle fiber will encounter a maternally or paternally inherited chromosome, this can produce a unique combination of maternal and paternal chromosomes that will make their way into the gametes.
In Anaphase I, the spindle fibers pull the linked chromosomes apart, while the sister chromatids remain tightly bound together at the centromere.
In Telophase I, the separated chromosomes arrive at opposite poles, after which the cells may separate or not, depending on the species.
Comparing Meiosis and Mitosis
Mitosis and meiosis are both forms of division of the nucleus in eukaryotic cells. They share some similarities, but also exhibit distinct differences that lead to very different outcomes. Mitosis is a single nuclear division that results in two nuclei that are usually partitioned into two new cells. The nuclei resulting from a mitotic division are genetically identical to the original nucleus, with the same number of sets of chromosomes. In contrast, meiosis consists of two nuclear divisions resulting in four nuclei that are partitioned into four new cells. The nuclei resulting from meiosis are not genetically identical and they contain one chromosome set only.
Meiosis II
In some species, cells enter a brief interphase, or interkinesis, before entering meiosis II, but no S phase, nor chromosomal duplication, will take place.
In Prophase II, if the chromosomes decondensed in telophase I, they condense again. If nuclear envelopes were formed, they fragment into vesicles. The centrosomes duplicated during interkinesis move away from each other toward opposite poles, and new spindles are formed. Each sister chromatid attaches to microtubules from opposite poles.
In Metaphase II, the sister chromatids are aligned at the equator of the cell.
In Anaphase II, sister chromatids are pulled apart by the spindle fibers, move toward opposite poles.
Finally, in telophase II and cytokinesis, the chromosomes arrive at opposite poles and, and nuclear envelopes form. Cytokinesis separates the two cells into four unique haploid cells. The cells produced are genetically unique because of the random assortment of paternal and maternal homologs and because of the recombining of maternal and paternal segments of chromosomes (with their sets of genes) that occurs during crossover.
To get a better sense of the process of meiosis, view the video linked below. You can watch it at full speed with the play button, or click each of the phases listed on the right to click through them one at a time. Pay special attention to the way in which genetic diversity is created during Prophase I, and also the number of chromosomes that are present in each of the resulting daughter cells at the end of Meiosis II.
https://www.cellsalive.com/meiosis_js.htm
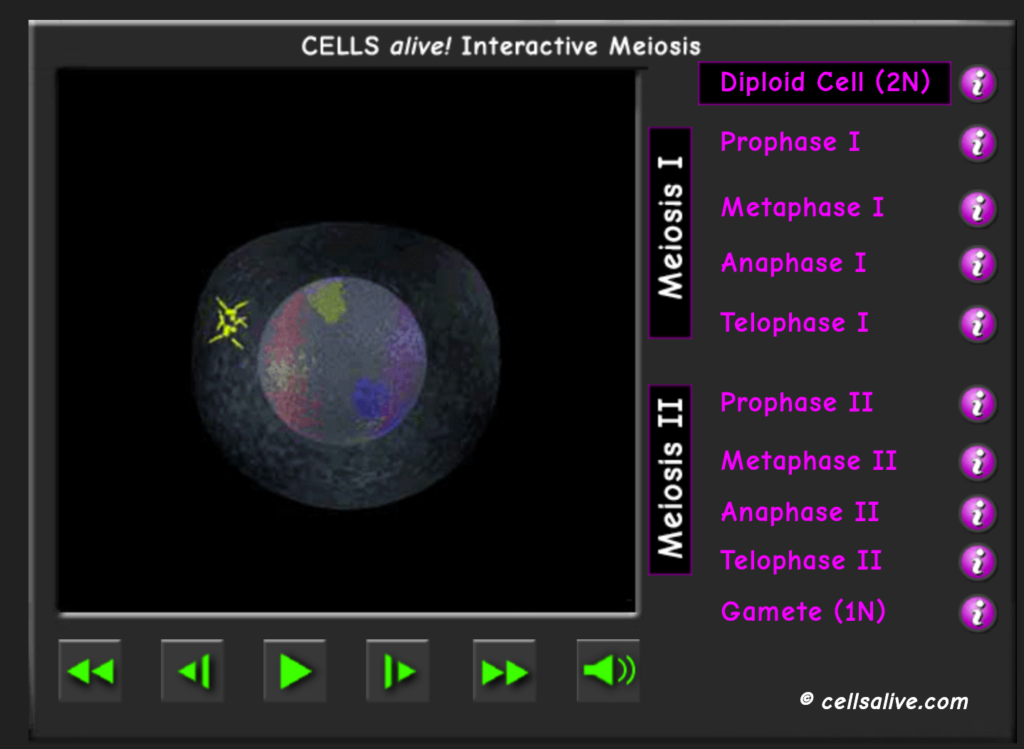
The following definitions may be useful:
- Oogonium: an immature female reproductive cell that gives rise to primary oocytes (egg cells)
- Spermatogonium: a cell produced at an early stage in the formation of spermatozoa (sperm cells)
- Sister chromatids: identical copies formed by the DNA replication of a chromosome, with both copies joined together by a common centromere
- Homologous dyads: a pair of homologous chromosomes (also known as sister chromatids)
- Tetrad: the four cells produced by meiotic cell division
- Chiasma: a point at which paired chromosomes remain in contact during the first metaphase of meiosis, and at which crossing over and exchange of genetic material occur between the strands
- Kinetochore: a complex of proteins associated with the centromere of a chromosome during cell division, to which the microtubules of the spindle attach
- Ploidy (tetraploid vs diploid vs haploid): the number of sets of chromosomes in a cell (four vs two vs one)
Inheritance
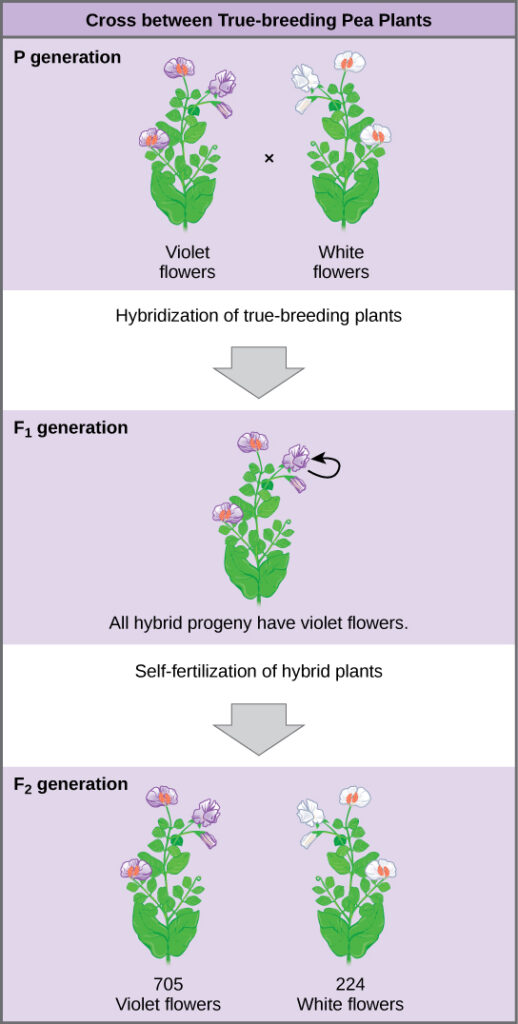
Genetics is the study of heredity. Genes, carried on chromosomes, are the basic functional units of heredity with the capability to be replicated, expressed, or mutated.
Johann Gregor Mendel (1822–1884) was an Augustinian monk who researched patterns of inheritance in pea plants. He demonstrated that some traits are transmitted faithfully from parents to offspring in predictable patterns. Mendel performed hybridizations, which involve mating two true-breeding individuals (those that always produce offspring that look like the parent), and tracking the phenotypes (appearance) of subsequent generations. His research demonstrated that there are different versions of genes – what we now call alleles – that determine the traits offspring will have.
Some terms to understand:
- Character – an observable feature (for example, hair color)
- Trait – a variation of a character (for example, blonde, brunette, red)
- Allele – a version of a gene
- Genotype – the combination of alleles that an organism inherited (one from each parent)
- Phenotype – the way the genotype is expressed, usually thought of as the physical appearance for the character in question
- Homozygote – the condition of having inherited two copies of the same allele at a given gene or locus
- Heterozygote – the condition of having inherited two different alleles at a given gene or locus
Not all genes are transmitted from parents to offspring according to Mendelian genetics, but Mendel’s experiments serve as an excellent starting point for thinking about inheritance.
Phenylthiocarbamide
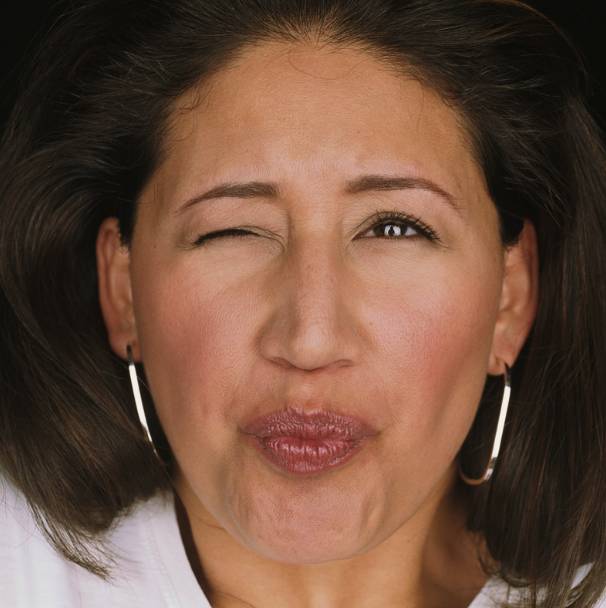
In 1931, a chemist named Arthur Fox was pouring some powdered phenylthiocarbamide (PTC) into a bottle. When some of the powder accidentally blew into the air, a colleague standing nearby complained that the dust tasted bitter. Fox tasted nothing at all. Curious how they could be tasting the chemical differently, they tasted it again. The results were the same. Fox had his friends and family try the chemical then describe how it tasted. Some people tasted nothing. Some found it intensely bitter, and still others thought it tasted only slightly bitter.
Soon after its discovery, geneticists determined that there is an inherited component that influences how we taste PTC. Today we know that the ability to taste PTC (or not) is conveyed by a single gene that codes for a taste receptor on the tongue. The PTC gene, TAS2R38, was discovered in 2003. There are two common alleles (versions of a gene) of the PTC gene, and at least five rare forms. One of the common forms is a tasting allele, and the other is a non-tasting allele. Each allele codes for a bitter taste receptor protein with a slightly different shape. The shape of the receptor protein determines how strongly it can bind to PTC. Since all people have two copies of every gene, combinations of the bitter taste gene variants determine whether someone finds PTC intensely bitter, somewhat bitter, or without taste at all. The ratio of tasters to non-tasters varies between populations, but every group has some tasters and some non-tasters. On average, 75% of people can taste PTC, while 25% cannot.
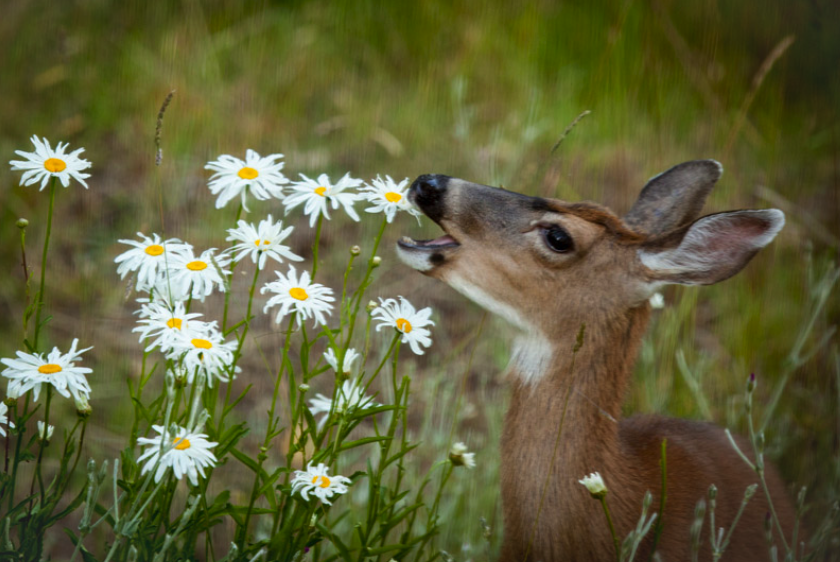
Although PTC is not found in nature, the ability to taste it correlates strongly with the ability to taste other bitter substances that do occur naturally, many of which are toxins. Plants produce a variety of toxic compounds in order to protect themselves from being eaten. The ability to discern bitter tastes evolved as a mechanism to prevent early humans from eating poisonous plants – those humans who had the ability to avoid toxic plants were better able to survive and pass on their alleles to offspring. Humans have about 30 genes that code for bitter taste receptors. Each receptor can interact with several compounds, allowing people to taste a wide variety of bitter substances. Plants are much more likely than animals to contain toxins. Because avoiding bitter plants would severely limit their food sources, strict herbivores have fewer bitter taste genes than omnivores or carnivores. Instead, animals that graze on plants have a high tolerance to toxins. Grazers have large livers that can break down toxic compounds.
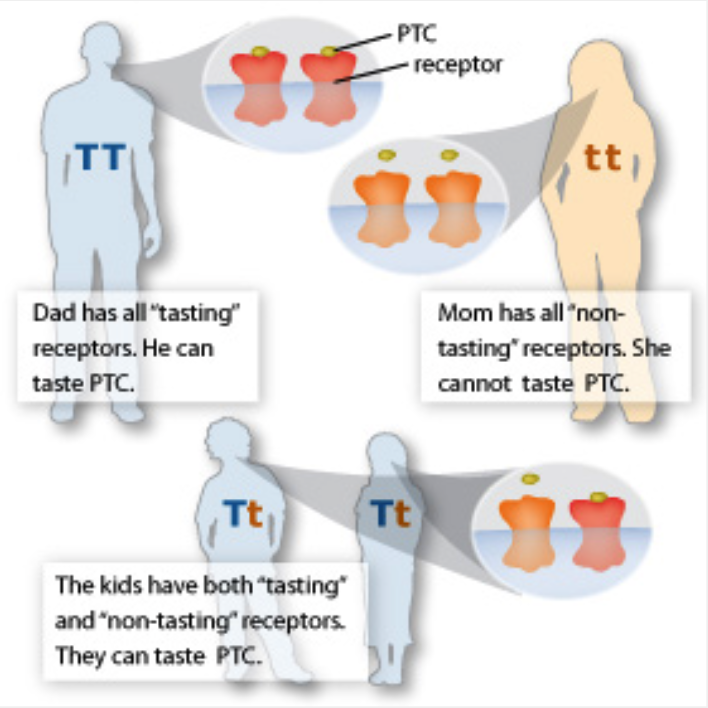
If the ability to taste bitter compounds conveys a selective advantage, then shouldn’t non-tasters have died off long ago? Why do so many people still carry the non-tasting PTC variant? Some scientists believe that non-tasters of PTC can taste another bitter compound. This scenario would give the greatest selective advantage to heterozygotes, or people who carry one tasting allele and one non-tasting allele. The ability to taste PTC shows a dominant pattern of inheritance. A single copy of a tasting allele (T) conveys the ability to taste PTC. Non-tasters have two copies of a non-tasting allele (t).
The chemical structure of PTC resembles toxic alkaloids found in some poisonous plants, like ricin, which can be extracted from castor beans. It may also be the reason that some people find broccoli and cabbage bitter to the taste.
The PTC gene is one example of an inherited trait in humans that is determined by a single gene. Today, we’ll see which allele of this gene you possess.
Heredity
For centuries humans have selected plant varieties and animals for specific traits.
- Plant breeders select plant varieties which produce more seed or fruit
- Livestock producers select animals with specific traits such as increased milk production, ample muscle mass or structural correctness
- Dogs have been bred for physical traits like eyesight and speed, as well as personality traits such as aggression or friendliness
Heredity is the passing on of traits from parents to offspring. Most plants and animals have two of every kind of gene, one from their mother and one from their father.
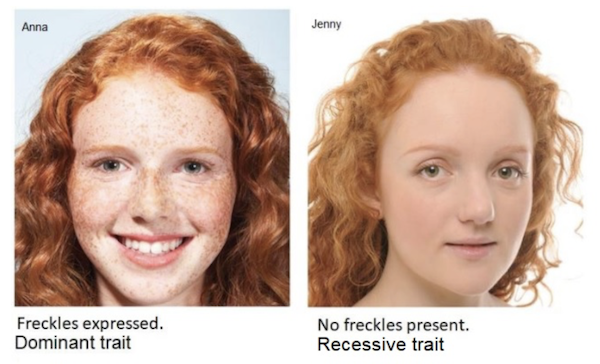
- Only one allele from each parent is passed to each offspring for a particular trait.
- Alleles are forms of the same gene with small differences in their DNA sequence. These small differences contribute to each organism’s unique physical features.
- These physical features are called phenotypes.
- The genetic makeup of an organism is its genotype.
Some alleles are dominant while others are recessive.
- Dominant alleles are always expressed in offspring
- Recessive alleles are only expressed in offspring if both parents contribute a recessive allele
For example, in humans, the allele for freckles is dominant.
- Children who receive a “freckle” allele from either parent will exhibit the trait
- Children who receive two recessive alleles will be freckle-less.
Text adapted from “Biology” by Openstax College. Download for free at: http://cnx.org/content/col11448/latest/